Advancing cancer research using bioprinting for tumor-on-a-chip platforms
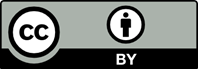
There is an urgent for a novel approach to cancer research with 1.7 million new cases of cancer occurring every year in the United States of America. Tumor models offer promise as a useful platform for cancer research without the need for animal models, but there remains a challenge to fabricate a relevant model which mimics the structure, function and drug response of human tumors. Bioprinting can address this need by fabricating three-dimensional constructs that mimic tumor heterogeneity, vasculature and spheroid structures. Furthermore, bioprinting can be used to fabricate tissue constructs within microfluidic platforms, forming “tumor-on-a-chip” devices which are ideal for high-throughput testing in a biomimetic microenvironment. Applications of tumors-on-a-chip include facilitating basic research to better understand tumor development, structure and function as well as drug screening to improve the efficiency of cancer drug discovery.
1. Cancer statistics n.d., viewed February 9, 2015, http://www.cancer.gov/about-cancer/what-is-cancer/statistics
2. Ridky T W, Chow J M, Wong D J, et al. 2010, Invasive three-dimensional organotypic neoplasia from multiple normal human epithelia. Nature Medicine, vol.16(12): 1450–1455. http://dx.doi.org/10.1038/nm.2265
3. Kim B J, Hannanta-anan P, Chau M, et al. 2013, Cooperative roles of SDF-1α and EGF gradients on tumor cell migration revealed by a robust 3D microfluidic model. PLoS One, vol.8(7): e68422. http://dx.doi.org/10.1371/journal.pone.0068422
4. Loessner D, Stok K S, Lutolf M P, et al. 2010, Bioengineered 3D platform to explore cell-ECM interactions and drug resistance of epithelial ovarian cancer cells. Biomaterials, vol.31(32): 8494–8506. http://dx.doi.org/10.1016/j.biomaterials.2010.07.064
5. Zhao Y, Yao R, Ouyang L, et al. 2014, Three-dimensional printing of Hela cells for cervical tumor model in vitro. Biofabrication, vol.6(3): 035001. http://dx.doi.org/10.1088/1758-5082/6/3/035001
6. Friedl P and Wolf K, 2010, Plasticity of cell migration: A multiscale tuning model. Journal of Cell Biology, vol.188(1): 11–19. http://dx.doi.org/10.1083/jcb.200909003
7. Li C L, Tian T, Nan K J, et al. 2008, Survival advantages of multicellular spheroids vs. monolayers of HepG2 cells in vitro. Oncology Reports, vol.20(6): 1465–1471. http://dx.doi.org/10.3892/or_00000167
8. Chopra V, Dinh T V and Hannigan E V, 1997, Three-dimensional endothelial-tumor epithelial cell interac-tions in human cervical cancers. In Vitro Cellular & Developmental Biology-Animal, vol.33(6): 432–442. http://dx.doi.org/10.1007/s11626-997-0061-y
9. Knowlton S, Onal S, Chu H Y, et al. 2015, Bioprinting for cancer research. Trends in Biotechnology, vol.33(9): 504–513. http://dx.doi.org/10.1016/j.tibtech.2015.06.007
10. Huh D, Hamilton G A and Ingber D E, 2011, From 3D cell culture to organs-on-chips. Trends in Cell Biology, vol.21(12): 745–754. http://dx.doi.org/10.1016/j.tcb.2011.09.005
11. Huh D, Torisawa Y S, Hamilton G A, et al. 2012, Microengineered physiological biomimicry: organs-on- chips. Lab on a Chip, vol.12(12): 2156–2164. http://dx.doi.org/10.1039/c2lc40089h
12. Chang S F, Chang C A, Lee D-Y, et al. 2008, Tumor cell cycle arrest induced by shear stress: roles of integrins and Smad. Proceedings of the National Academy of Sciences of the United States of America, vol.105(10): 3927–3932. http://dx.doi.org/10.1073/pnas.0712353105
13. Polacheck W J, Charest J L and Kamm R D, 2011, Interstitial flow influences direction of tumor cell migration through competing mechanisms. Proceedings of the National Academy of Sciences of the United States of America, vol.108(27): 11115–11120. http://dx.doi.org/10.1073/pnas.1103581108
14. Varani J, 1982, Chemotaxis of metastatic tumor cells.
Cancer Metastasis Reviews, vol.1(1): 17–28. http://dx.doi.org/10.1007/BF00049478
15. Ghaemmaghami A M, Hancock M J, Harrington H, et al. 2012, Biomimetic tissues on a chip for drug discovery. Drug Discovery Today, vol.17(3–4): 173–181. http://dx.doi.org/10.1016/j.drudis.2011.10.02
16. Dababneh A B and Ozbolat I T, 2014, Bioprinting technology: A current state-of-the-art review. Journal of Manufacturing Science and Engineering, vol.136(6): 061016. http://dx.doi.org/10.1115/1.4028512
17. Junttila M R and de Sauvage F J, 2013, Influence of tumour micro-environment heterogeneity on therapeutic response. Nature, vol.501(7467): 346–354. http://dx.doi.org/10.1038/nature12626
18. Xu F, Celli J, Rizvi I, et al. 2011, A three-dimensional in vitro ovarian cancer coculture model using a high-throughput cell patterning platform. Biotechnology Journal, vol.6(2): 204–212. http://dx.doi.org/10.1002/biot.201000340
19. Weiss L E, Amon C H, Finger E D, et al. 2005, Bayesian computer-aided experimental design of heterogeneous scaffolds for tissue engineering. Computer-Aided Design, vol.37(11): 1127–1139. http://dx.doi.org/10.1016/j.cad.2005.02.004
20. Keenan T M and Folch A, 2008, Biomolecular gradients in cell culture systems. Lab on a Chip, vol.8(1): 34–57. http://dx.doi.org/10.1039/B711887B
21. Nagy J A, Chang S H, Dvorak A M, et al. 2009, Why are tumour blood vessels abnormal and why is it important to know? British Journal of Cancer, vol.100(6): 865–869. http://dx.doi.org/10.1038/sj.bjc.6604929
22. Huang T Q, Qu X, Liu J, et al. 2014, 3D printing of biomimetic microstructures for cancer cell migration. Biomedical Microdevices, vol.16(1): 127–132. http://dx.doi.org/10.1007/s10544-013-9812-6
23. Chauhan V P, Stylianopoulous T, Martin J D, et al. 2012, Normalization of tumour blood vessels improves the de-livery of nanomedicines in a size-dependent manner. Nature Nanotechnology, vol.7(6): 383–388. http://dx.doi.org/10.1038/nnano.2012.45
24. Hirschhaeuser F, Menne H, Dittfeld C, et al. 2010, Multicellular tumor spheroids: an underestimated tool is catching up again. Journal of Biotechnology, vol.148(1): 3–15. http://dx.doi.org/10.1016/j.jbiotec.2010.01.012
25. Hribar K C, Finlay D, Ma X, et al. 2015, Nonlinear 3D projection printing of concave hydrogel microstructures for long-term multicellular spheroid and embryoid body culture. Lab on a Chip, vol.15(11): 2412–2418. http://dx.doi.org/10.1039/c5lc00159e
26. Vidi P A, Maleki T, Ochoa M, et al. 2014, Disease-on-a-chip: mimicry of tumor growth in mammary ducts. Lab on a Chip, vol.14(1): 172–177. http://dx.doi.org/10.1039/c3lc50819f
27. Rizvi I, Gurkan U A, Tasoglu S, et al. 2013, Flow induces epithelial-mesenchymal transition, cellular heterogeneity and biomarker modulation in 3D ovarian cancer nodules. Proceedings of the National Academy of Sciences, vol.110(22): E1974–E1983. http://dx.doi.org/10.1073/pnas.1216989110
28. Albanese A, Lam A K, Sykes E A, et al. 2013, Tumour-on-a-chip provides an optical window into nanoparticle tissue transport. Nature Communications, vol.4: 2718. http://dx.doi.org/10.1038/ncomms3718
29. Kwak B, Ozcelikkale A, Shin C s, et al. 2014, Simulation of complex transport of nanoparticles around a tumor using tumor-microenvironment-on-chip. Journal of Controlled Release, vol.194: 157–167. http://dx.doi.org/10.1016/j.jconrel.2014.08.027
30. Chang R, Nam J and Sun W, 2008, Direct cell writing of 3D microorgan for in vitro pharmacokinetic model. Tissue Engineering Part C Methods, vol.14(2): 157–166. http://dx.doi.org/10.1089/ten.tec.2007.0392
31. Snyder J, Son A R, Hamid Q, et al. 2015, Fabrication of microfluidic manifold by precision extrusion deposition and replica molding for cell-laden device. Journal of Manufacturing Science and Engineering, vol.138(4): 041007. http://dx.doi.org/10.1115/1.4031551
32. Hamid Q, Wang C, Zhao Y, et al. 2014, A three-dimensional cell-laden microfluidic chip for in vitro drug metabolism detection. Biofabrication, vol.6(2): 025008. http://dx.doi.org/10.1088/1758-5082/6/2/025008
33. Hamid Q, Wang C, Synder J, et al. 2015, Maskless fabrication of cell-laden microfluidic chips with localized surface functionalization for the co-culture of cancer cells. Biofabrication, vol.7(1):015012. http://dx.doi.org/10.1088/1758-5090/7/1/015012
34. An Uphill Battle, n.d., viewed February 18, 2016, http://www.brightfocus.org/sites/default/files/An%20Uphill%20Battle.jpg
35. PR Tufts CSDD 2014 Cost Study, 2014, viewed Febru-
ary 18, 2016, <http://csdd.tufts.edu/news/complete_story/pr_tufts_csdd_2014_cost_study>
36. Unger C, Kramer N, Walzl A, et al. 2014, Modeling human carcinomas: Physiologically relevant 3D models to improve anti-cancer drug development. Advanced Drug Delivery Reviews, vol.79–80: 50–67. http://dx.doi.org/10.1016/j.addr.2014.10.015
37. Singh M, Morris C P, Ellis R J, et al. 2008, Micro-sphere-based seamless scaffolds containing macroscopic gradients of encapsulated factors for tissue engineering. Tissue Engineering Part C—Methods, vol.14(4): 299–309. http://dx.doi.org/10.1089/ten.tec.2008.0167
38. He Z Q and Xiong L Z, 2011, Fabrication of poly(D, L-lactide-co-glycolide) microspheres and degradation characteristics in vitro. Journal of Macromolecular Science Part B—Physics, vol.50(9): 1682–1690. http://dx.doi.org/10.1080/00222348.2010.543036
39. Bertassoni L E, Cardoso J C, Manoharan V, et al. 2014, Direct-write bioprinting of cell-laden methacrylated gelatin hydrogels. Biofabrication, vol.6(2): 024105. http://dx.doi.org/10.1088/1758-5082/6/2/024105
40. Tasoglu S and Demirci U, 2013, Bioprinting for stem cell research. Trends in Biotechnology, vol.31(1): 10–19. http://dx.doi.org/10.1016/j.tibtech.2012.10.005
41. Durmus N G, Tasoglu S and Demirci U, 2013, Bio-printing: functional droplet networks. Nature Materials, vol.12(6): 478–479. http://dx.doi.org/10.1038/nmat3665
42. Ozbolat I T and Yu Y, 2013, Bioprinting toward organ fabrication: challenges and future trends. IEEE Transactions on Biomedical Engineering, vol.60(3): 691–699. http://dx.doi.org/10.1109/TBME.2013.2243912
43. Kolesky D B, Truby R L, Gladman A S, et al. 2014, 3D bioprinting of vascularized, heterogeneous cell-laden tissue constructs. Advanced Materials, vol.26(19): 3124– 3130. http://dx.doi.org/10.1002/adma.201305506
44. Drug Discovery, 2015, InvivoSciences, viewed February 18, 2016, http://invivosciences.com/products-services/drug-discovery/